Meet Dr. David Harrison
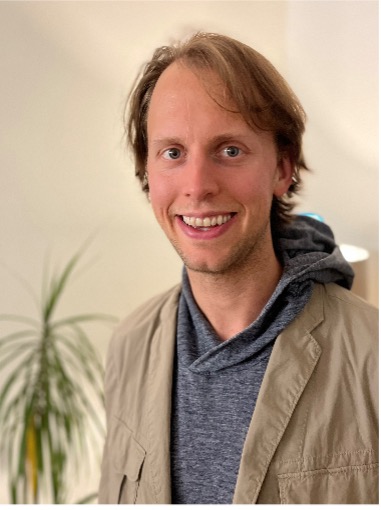
Dr. David Harrison
Advisor: Dr. Robert F. McDermott
Institution: University of Wisconsin–Madison
Bio: David Harrison was born and raised in central Minnesota, where he earned his bachelor’s degree at St. John's University. After college, he worked for several years in software development. In that work, he realized that the thing he enjoyed the most—and was best at—was helping his colleagues learn. With an eye on a future teaching career, he entered graduate school and earned a Ph.D. in Physics from the University of Minnesota in January 2021. In his research there, he used electronic noise in mesoscale spin-glasses—a type of disordered magnet—as a probe of their underlying magnetic state. After completing his doctorate, he taught as a Visiting Assistant Professor at Carleton College in Winter 2021, before beginning an IC Postdoctoral Fellowship at University of Wisconsin-Madison. The focus of his fellowship work is the resonant absorption of pair-breaking radiation in superconducting qubits, work which also has applications in next-generation dark matter detectors.
Abstract: Superconducting qubits are resonant absorbers of pair-breaking radiation, as the qubit structure is the aperture dual of a wire loop antenna. Typical Josephson junction parameters result in a junction impedance that is reasonably well matched to the fundamental antenna mode. This spurious antenna mode contributes to nonequilibrium quasiparticle poisoning in superconducting qubits; with a careful understanding of this mode, we can implement mitigation strategies by varying the device scale, exploring highly symmetrized designs, and by gap engineering to push the gap above the antenna mode. We can exploit the same physics to realize a new class of quantum sensors for mm-wave radiation based on the resonant transduction of pair-breaking photons to quasiparticles. We use broadband thermal radiation and coherent mm-wave radiation derived from a Josephson photon source to characterize these detectors over the band from 100 GHz to 1 THz; the bandwidth can be extended with gap engineering. We have measured upward and downward transition rates and charge-parity rates as a function of radiator temperature or coherent drive frequency. In addition, we are characterizing detectors coupled to microwave kinetic inductance detectors (MKIDs). These schemes could form the basis for next-generation detectors of dark-matter axions, in a mass range difficult to access by other means.